Krystal Pynn1, Chung-Ying Tsai
2,3, and Alicia M. Koontz
2,3
- Department of Biomedical Engineering, Stony Brook University, Stony Brook, NY
- Human Engineering Research Laboratories, VA Pittsburgh Healthcare System, Pittsburgh, PA
- Department of Rehabilitation Science and Technology, University of Pittsburgh, Pittsburgh, PA
Introduction
For a manual wheelchair user, proper wheelchair transfer techniques can make the difference between a healthy, independent lifestyle and one with increased onsets of pain and limited mobility. A sitting pivot transfer (SPT) is the most common type of transfer performed by wheelchair users who are unable to stand unsupported. On average, wheelchair users perform around 15 to 20 SPTs daily in order to transfer to a commode, bed, car or other surfaces of equal or varying height (Finley, McQuade, & Rodgers, 2005) . However, many manual wheelchair users complain of shoulder pain with transfers (Alm, Saraste, & Norrbrink, 2008). The high reliance on the upper limbs to lift and support the body weight while pivoting to the target surface is believed to be partially responsible for the high prevalence of shoulder pain (Dalyan, Cardenas, & Gerard, 1999). The resulting pain results in activity limitations, increased health care expenses and depression.
Current literature suggests that certain movement strategies used during transfers have the potential to be damaging to musculoskeletal tissues (Boninger, 2013). For example, the combination of extraneous shoulder flexion, abduction and internal rotation combined with weight-bearing loads at the shoulders may result in musculoskeletal injuries to the rotator cuff (Allison, Singer, & Marshall, 1996). Maintaining a trunk upright posture throughout the transfer motion also increases the magnitude of vertically-directed loads acting at the shoulder (Koontz, Kankipati, Lin, Cooper, & Boninger, 2011). High vertically-directed loading at the shoulder during wheelchair activities such as weight relief raises, propulsion and transfers are believed to cause compression of the subacromial space and result in impingement of soft tissues and bursae (Gagnon et al., 2009).
Recent studies suggest that there are certain movement strategies that may help reduce forces and extraneous joint movements (Koontz et al., 2011). Positioning the leading hand close to the body during level bench transfers was found to result in a more even distribution of forces across the upper limb joints whereas a hand placed further way increased the peak resultant forces on the trailing arm (Kankipati, 2012). Research also suggests that forward trunk flexion during transfers helps to engage larger muscles around the shoulder (e.g. sternal pectoralis major and latissimus dorsi muscle groups) allowing for less loading of the glenohumeral joint thereby reducing the risk of rotator cuff impingement (Perry, Gronley, Newsam, Reyes, & Mulroy, 1996).
The Transfer Assessment Instrument (TAI) is a scoring system that serves as a guideline for clinicians to determine if a patient is transferring using the best techniques according to research and to aid in identifying specific problems with the transfer process. For example, using the TAI assessment results, clinicians can identify and quantify if appropriate wheelchair setup, hand, arm and trunk positioning, and smooth and controlled body movements are being used. This information can then be used to design an individualized transfer training program that addresses the deficits in the transfer technique (Rice et al., 2013). The TAI has high inter- and intra- rater reliability (McClure, Boninger, Ozawa, & Koontz, 2011; Tsai, Rice, Hoelmer, Boninger, & Koontz, 2013) and aligns well with expert clinicians' opinions of proper technique (Boninger et al., 2005).
An important general concept with transfers is that the person position their rear or trailing hand next to their body and rearward and their forward or leading hand in front of and away from the body (Minkel, Hastings, McClure, & Bjerkefors, 2010). This hand placement facilitates the pivot action of the lower body, which needs to move in opposition to the upper body. This pivot action is often referred to as the head-hips relationship (Minkel et al., 2010). As mentioned, prior transfer biomechanics research has shown that placing the leading hand further out from the body during transfers increases joint loading (Kankipati, 2012). The purpose of this study was to assess TAI's sensitivity to detecting changes in the quality of transfer as a function of hand placement and gain a greater understanding of how quality of transfer movement is impacted by hand positioning relative to the trunk. The findings of the study will generate data that can be used to help define appropriate movement strategies for wheelchair to toilet SPTs and data that further supports the construct validity of the TAI.
METHODS
Subjects
The study was approved by the Department of Veterans Affairs Institutional Review Board. Subjects were recruited from an IRB approved research registry and signed an approved informed consent document before performing any test procedures. The inclusion criteria were: 1) have discernible neurological impairment affecting both lower extremities or a double transfemoral or transtibial amputation, 2) at least one year post diagnosis or injury, 3) use a wheelchair for mobility for over 40 hours per week, 4) able to sit upright for at least four hours a day, 5) over 18 years of age 6) able to independently transfer to and from a wheelchair without human assistance or an assistive device.
Experimental Protocol
Subjects positioned their wheelchairs on a custom designed transfer station (Figure 1). The transfer station included three force plates (Bertec Corporation, Columbus, OH), which were placed underneath the wheelchair, the toilet, and the subject’s feet, and two 6-component load cells (Model MC5 from AMTI, Watertown, MA; Model Omega 160 from ATI, Apex, NC), which were each attached to a steel beam used to simulate an armrest and grab bar (Figure 1). The grab bar was designed and positioned according to ADAAG guidelines concerning grab bar height and distance away from the toilet in accessible bathrooms ("Americans with Disabilities Act (ADA) - Accessibility Guidelines for Buildings and Facilities,"). The wheelchair and toilet were secured to the aluminum platform to prevent slippage during testing. Reflective markers were placed on anatomical landmarks of the subject’s trunk and upper extremities (Wu et al., 2005) . The markers’ coordinate positions were tracked by a ten-camera three-dimensional motion capture system (Vicon, Centennial, CO).
Before performing any transfers, subjects were asked to sit with their arms in an anatomical neutral position to collect a static trial of the marker positions. Afterwards, they were asked to transfer to the commode using their habitual approaches. Subjects were free to adjust their wheelchair orientation with respect to the commode within the confines of the space available on the platform (91.44cm by 91.44cm). Subjects performed up to five trials for each transfer. The subjects were restricted to moving to their left side from the wheelchair to the toilet based on the experimental setup. On the wheelchair side subjects were restricted to placing their (trailing) hand on the steel beam so forces during the transfer could be recorded. Subjects had the option to place their lead hand wherever they so desired on the toilet or the grab bar that was next to the toilet (Figure 1). Kinetic data from the force plates and load cell were collected at 1000 Hz while the kinematics data were collected at 100 Hz for the entire duration of the transfer.
During every transfer, the TAI was completed twice for the transfer to the toilet and back to the wheelchair. Two study clinicians trained on the TAI independently observed and scored each transfer. Two clinicians were used so as to reach consensus for any mismatch that may have occurred in item scoring.
Data Analysis
A zero-lag low-pass Butterworth 4th order filter with a cutoff frequency of 5 and 7 Hz was used to filter the kinect and kinematic data respectively in Matlab (Mathworks Inc., Natwick, MA, USA). Force data were downsampled to 100 Hz to match the sampling frequency of the kinematic data. The beginning and end of the transfers were determined using the vertical reaction forces from the load cell and the force plates underneath the toilet. The transfer began when the load cell detected the hand force (rising from zero). The end of the transfer was the moment before the toilet side force plate detected the landing spike of the buttocks (Kankipati, Koontz, Vega, & Lin, 2011). The lift phase began as soon as the buttocks lifted off the wheelchair and ended when the trunk was at its highest elevated point as indicated by the peak in the C7 vertebrae & T3 vertebrae position data (Kankipati et al., 2011). The descent phase was determined to be the time between the peak height of the C7 marker and when the buttocks landed on the toilet.
To determine hand position relative to the body we calculated the maximum distance between the leading (left) 3rd metatarsophalangeal joint (MCP) marker and the center of mass of the trunk (TCOM) during the entire transfer process and each transfer phase separately (e.g. lift and descent). The X, Y and Z-axis of the global coordinate reference system (GCS) are facing forward, leftward and upward, respectively (Figure 1). The TCOM was calculated using the averaged marker position of the left and right acromioclavicular joints (LAC & RAC) and the xiphoid process with respect to the GCS (Winter, 2009). The L3MCP-TCOM resultant distance (D) was computed using the following equation:
D=((MCPx-TCOM
x)
2+(MCP
y-TCOM
y)
2 + (MCP
z-TCOM
z)
2)
.5
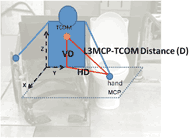
We also separately analyzed the vertical distance component (VD), which was the distance along the pure Z direction, and a horizontal distance component (HD), which was equivalent to the hypotenuse of the X- and Y-component distances and located in the transverse plane (Figure 2). A Pearson correlation test was used to test the association between L3MCP-TCOM resultant distance (D) and the arm length. This association was not statistically significant (p = 0.47) and the strength of the relationship was poor (r = 0.16) and thus the effects of subject anthropometry on the hand position data was presumed to be negligible.
The TAI contains two parts and three summary scores, which are the part 1 score, part 2 score, and the final score. Part 1 is comprised of 15 items and scored “Yes” which is 1 point, “No” which is 0 points, or not applicable “(N/A)” which means a removed item. The part 1 score is the summation of each item’s score multiplied by 10, and then divided by the number of applicable items, ranging from 0 to 10 (McClure et al., 2011). All of the items in Part 1 are completed after each transfer, and the scores for every transfer trial are averaged together (2 scores in this study) to produce a single representative item score. The 12 items in part 2 are scored on a Likert Scale ranging from 0 to 4. A ‘0’ means strongly disagree, and ‘4’ means strongly agree. The part 2 score is the summation of each item’s score multiplied by 2.5, and then divided by the number of applicable items, resulting in a range of scores from 0 to 10. The items in part 2 are completed after all transfers trials have been performed. The final score of the TAI is the average of the part 1 and part 2 scores.
Correlation test statistics in SPSS (SPSS Inc., Chicago) were used to assess the association between the Vicon computed hand positioning variables, TAI summary scores, and TAI item scores that corresponded specifically to hand, arm and trunk positioning during transfers (Part 1 Items 8, 9, 12 and 13). All data were examined for normality using Shapiro-Wilk test and checked for outliers using Q-Q plot. Because some of the variables were not normally distributed and some were ordinal data (e.g. TAI item scores), we used the Spearman’s correlation coefficient. A p-value less than 0.05 was used to determine significant correlations.
RESULTS
Fourteen subjects volunteered to participate in the study. Eight of these subjects came back a second time to undergo transfer technique training as part of a larger study and their new transfer biomechanics data were collected on the same day. As their biomechanics were different post-training we included both datasets (eg. from their baseline and post training visits) in this analysis along with the six subjects who had only baseline transfer biomechanics testing. Therefore, there were a total 22 transfer data sets used in this analysis. The group mean (± standard deviation) of age, height and weight for the 14 subjects were 39 (± 11) years, 170.4 (± 9.4) centimeters and 67.9 (± 15.6) kilograms, respectively. Thirteen subjects had spinal cord injuries (SCI) with levels that ranged from C2 to L4. Nine subjects had a complete SCI and four subjects had an incomplete SCI. One subject had a bilateral tibial and fibular fracture with nerve damage. The average duration (± standard deviation) of using a wheelchair was 13 (± 8.5) years.
Table 1 shows the maximal distances computed between trunk COM and L3MCP over the entire transfer process (both lift and descent phases combined). Nine subjects placed their leading hand on the rim of the toilet when they transferred while the remaining subjects used the grab bar next to the toilet (Figure 1).
|
Max HD |
Max VD |
Max D |
---|---|---|---|
Mean ±SD (cm) | 52.11 ±16.75 | 44.07 ±12.94 | 68.56 ±11.67 |
TCOM = center of mass of trunk; L3MCP = Left 3rd Metacarpalphalangeal joint; Max = maximal; HD = horizontal distance; VD = vertical distance; D = resultant displacement; SD = standard deviation |
Table 2 shows that lower summary TAI scores (poorer quality transfers) on part 1 and final scores were significantly correlated to greater horizontal distances and displacements between the lead hand and trunk COM (e.g. r values ranged from -0.50 to -0.71, p < 0.05). Items 8, 9, 12 and 13 also showed a significant negative correlation with maximal hand-trunk displacement and the horizontal component distance during both the lift and descent phases with r values ranging from -0.45 to -0.59, p < 0.05 (Table 2). Higher scores on Item 13 in part 1 of TAI were significantly correlated (r=0.43 and 0.38 respectively, p < 0.05) to greater vertical height distances between the hand and trunk in both of the lift and descent phases (Table 2).
|
|
P1 |
Final |
Item 8 |
Item 9 |
Item 12 |
Item 13 |
---|---|---|---|---|---|---|---|
Lift |
Max HD | -0.69¶ | -0.71¶ | -0.37 | -0.55¶ | -0.55¶ | -0.45* |
Max VD | 0.32 | 0.35 | 0.32 | 0.33 | 0.10 | 0.43* | |
Max D | -0.58¶ | -0.61¶ | -0.49* | -0.53* | -0.47* | -0.52* | |
Descent |
Max HD | -0.50* | -0.53* | -0.50* | -0.51¶ | -0.39 | -0.56¶ |
Max VD | 0.22 | 0.25 | 0.28 | 0.27 | 0.03 | 0.38* | |
Max D | -0.57¶ | -0.59¶ | -0.58¶ | -0.55¶ | -0.42 | -0.59¶ | |
¶ Correlation is significant at p < 0.01 * Correlation is significant at p < 0.05 Max = Maximal; HD = Horizontal Distance; VD = Vertical Distance; D = Resultant Distance; |
DISCUSSION
This study found significant relationships between the quality of transfer movement and lead hand positioning during SPTs. The subjects in this study had the option to place their lead hand wherever they so desired and a majority of subjects (64%) chose to place their hand somewhere on the rim of the toilet seat rather than the grab bar next to the toilet. The decreased overall quality of transfer (summary part 1 and total scores) with increased resultant and horizontal distance between the leading hand and trunk suggests that reaching further out to use the grab bar may be biomechanically unsuitable for those who independently perform SPTs in commercially accessible bathrooms. Moreover, the relationship found between hand-trunk distance and quality of the transfer corresponds well to the previous biomechanical study that found forces increase when the lead arm is positioned further away from the body (Kankipati, 2012). An increase in force at the shoulder joint combined with extraneous arm positioning (e.g. a more abducted arm) potentially reduces the subacromial space and predisposes wheelchair users to shoulder impingement syndrome (Boninger et al., 2005).
The TAI items 8, 9 and 13 were also significantly related to the hand-trunk resultant and horizontal distances. Item 8 states that hands need to put in stable position and be close to the body during transfers. Item 9 checks that an appropriate handgrip within subjects’ base of support is used by the leading arm. Item 13 states that the lead arm should not be extremely internally rotated and should not be abducted over 45 degrees. Our data indicate that higher scores on these three items (e.g. better upper limb movement quality) corresponded with subjects who placed their hands closer to their trunk COM. Large shoulder flexion and adduction moments are required at the leading arm to maintain good control of the body and dynamic balance throughout the transfer process (Gagnon et al., 2009). Keeping the leading arm close to the body helps to reduce the moment arm thereby minimizing the amount of muscular effort needed to successfully perform the transfer (Minkel et al., 2010). Moreover placing the lead shoulder in some internal rotation with pronation of the forearm and fingers that point toward the front edge of the transfer surface helps to 'pre-rotate the upper trunk preparing it for rotation about the 'fixed' lower trunk (Minkel et al., 2010). Our data also showed that individuals who scored well on this item (Item 13: the lead arm is correctly positioned) had a greater vertical distance between the trunk COM and lead hand marker. Thus, when the leading hand is positioned close, it seems the trunk may not need to flex as much to complete the rotary motion.
Proper transfer skill also makes use of the head-hip relationship. This study found that subjects who scored well on TAI item 12 (e.g. proper usage of head-hip relationship during SPT) had shorter hand-trunk resultant distances during the lift phase. The head-hips relationship is characterized by increased trunk flexion as the user pivots forward, shifting the COM forward and downward and creating a moment to lift and move the hips in the opposite direction of the head (Allison et al., 1996). This rotational strategy reduces the vertical forces in all upper limb joints and recruits larger muscle groups, such pectoralis major and latissimus dorsi, to help move the body to the target surface (Koontz et al., 2011 and Perry et al.). This is an ideal strategy to use during transfers as recruitment of the larger muscle groups around the shoulder helps to protect the smaller rotator cuff muscles from excessive loading (Finley et al., 2005; Koontz et al., 2011) .
Based on these results and that the lead hand needs to placed some distance forward of and away from the body, we recommend that the hand be placed such that there is just enough space for the buttocks to land on the target surface. The leading arm close to the body improves the overall quality of the transfer (findings from this study), reduces the lever arm for the necessary shoulder flexion and adduction moments (Gagnon et al., 2009) and lowers forces in the trailing limb (Kankipati, 2012). The significant correlations between TAI scores (part 1 and final scores) and the leading hand-trunk distances indicate that the TAI can help identify where transfer skills may be lacking and where training is needed to help correct potentially harmful and improper movement strategies.
One limitation to this study is the small sample size. However as multiple trials from the same subjects (e.g. pre-post transfer training trials) were included this likely increased the ability to detect statistically significant correlations between the TAI and hand positioning variables. Subjects who were identified at the baseline visit as having poor hand placement were taught to use better hand placement as part of the transfer training protocol. Initial hand placement during transfers is also influenced by how close the wheelchair is positioned next to the surface. The device positioning and the lead hand position are mutually considered on the TAI. In the current study, subjects performed toilet transfers. Some wheelchair users do not use the toilet for routine bowel-bladder care. All of our subjects reported doing toilet transfers however the frequency of doing such transfers was not collected. The mock bathroom setup in our study may also have differed from what subjects were used to at home or in other places. Because wheelchair users encounter different transfer environments throughout their daily lives, the experimental setup was not considered to be a major factor impacting the results.
CONCLUSION
The results of the study suggest that wheelchair users who perform SPTs position their leading hand just far enough away on the target surface to allow for the body to land right next to it. The TAI results followed the results of previous biomechanical studies that showed positioning the hand closer reduces the moment arm and force. Thus in the absence of biomechanics, the TAI can help clinicians to identify individuals who are at risk for developing injuries due to improper transfer techniques.REFERENCES
Americans with Disabilities Act (ADA) - Accessibility Guidelines for Buildings and Facilities.
ACKNOWLEDGMENTS
This material is based upon work supported by the Department of Veterans Affairs (B7149I) and from the National Science Foundation ASPIRE Grant #1262670. The contents of this paper do not represent the views of the Department of Veterans Affairs or the United States Government.
Audio Version PDF Version